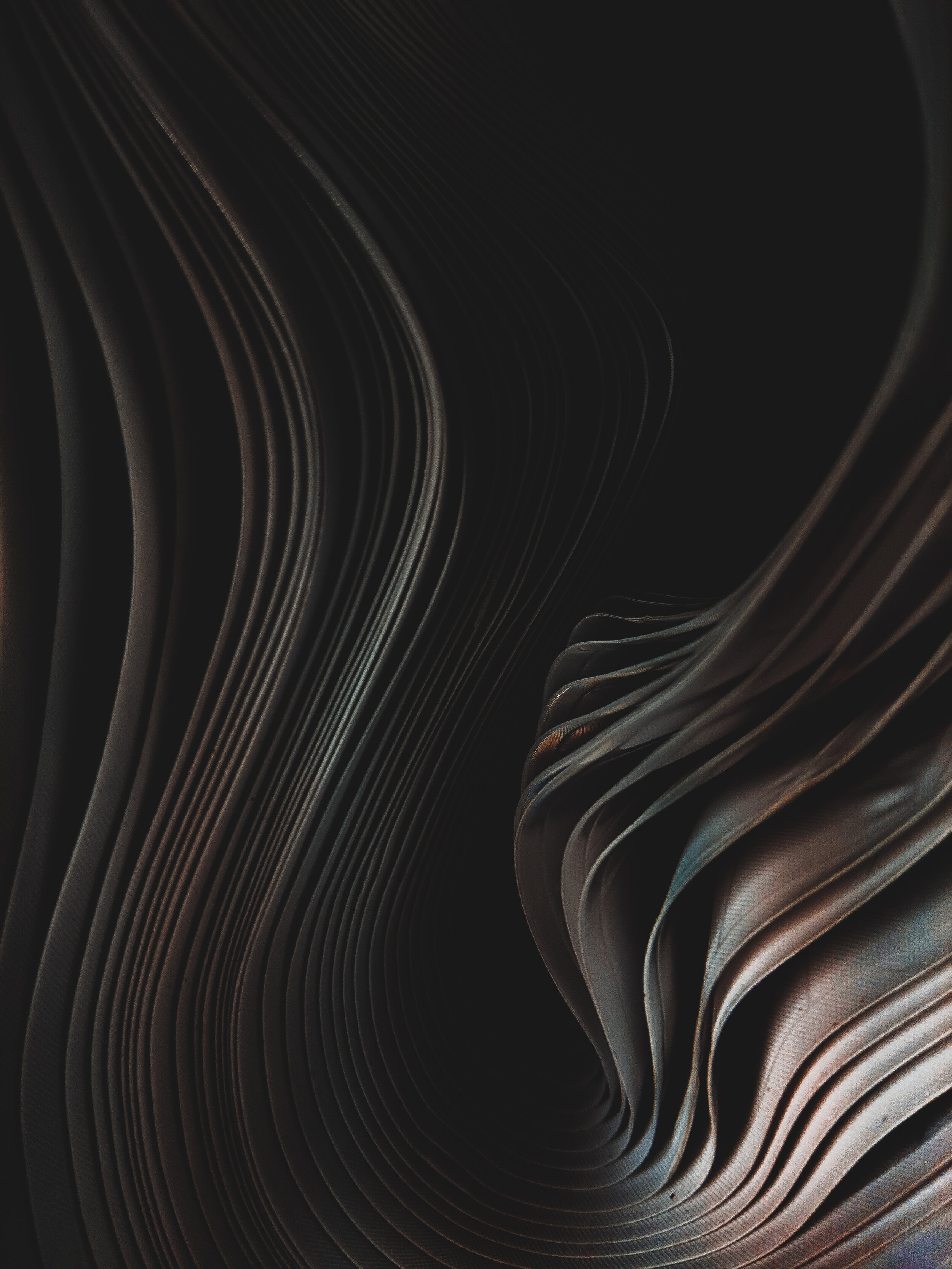
Facilities and Laboratories.
Nanoscale Electron Beam Lithography / Materials Processing / Dilution Cryostat / Superconducting Magnets / EMI and Acoustic Shielding Rooms / Wet Chemistry Labs / Multi-Physics Simulation / Shared Facilities
Facilities and Laboratories.
Fabrication, characterization and measurement of molecular-level nanostructures require facilities up to date with the current rate of progress of technology. Towards this end, we have set up extensive infrastructure for nanoscale fabrication and ultra-low temperature measurement to bio-functionalization of nanomechanical sensors. In addition to the in-house nanofabrication facilities, we have built a state-of-the-art ultra-sensitive laboratory with stealth capabilities, which allows a vibration-free, noise-free environment for measurements down to a low temperature of 0.006 Kelvin at GHz-range frequencies and picosecond timescales.
These labs are supported by truly cutting-edge facilities in both the department and the university. Engineering solutions in our projects often use the Scientific Instrumentation Facility (SIF) in our department for high-precision machining to large-scale structural designs. Other departmental facilities such as the Elctronic Design Facility (EDF) and high-performance (super-)computing and visualization facility are also instrumental to the work in our group. Perhaps the most essential is the Optical Processing Facility in the Photonics Center, which allows state-of-the-art processing techniques.
The following infrastructure is in existence now:
Ultra-Sensitive Low-Temperature Laboratory with DC-to-Daylight Noise Isolation
Acoustic Lab for Measurement of Nanomechanical Structures
Nanofabrication and Characterization Facility
Clean Room Facility for Bio-Functionalization
General Facilities in the Physics Department
Interdisciplinary Facilities in Boston University
Nanofabrication Facility.
Our in-house nanofabrication facility houses extensive capabilities from scanning e-beam writers to various materials processing systems, allowing fabrication of complex nanostructures. In addition to fabrication, growth and processing, the structures are characterized for both electrical continuity and structural integrity by a number of techniques. Integration of this facility with our measurement lab is important as it allows an efficient and effective approach to the projects which require everything from fabrication, processing, characterization to measurement in extreme environments.
The in-house nanofabrication facility spans an area of over 1500 sqft which has been remodeled to operate as a class-10,000 clean room. An isolated cleanroom inside the facility allows an even cleaner environment at the level of class 1000. A laminar-flow hood inside this clean room provides a volume of workspace with an environment down to class 100. A number of fume hoods and laminar-flow hoods provide localized clean space.
The types of nanostructures manufactured in our lab vary in their fabrication techniques, materials, and level of complexity. Nanoelectronics structures typically involve metallic nanowires or quantum dots on silicon substrates. Nanomechanical structures are suspended devices with three-dimensional relief with simple designs of beams and cantilevers to antennas and complex torsion oscillators. Some of these structures have many layers of materials to have provisions for access to local electrical, magnetic and/or mechanical signals. Bio-functionalized structures are nanomechanical structures with additional processes for coating biomaterials with nanometer scale resolution. Tip-enhanced microscopy requires metallic pillars or tips with a diamater of roughly 10 nm and a height of up to 1 micron. In addition to these structures, we are also developing techniques for high-density nanostructures by combining hard and soft lithography.
Electron-Beam Writer for Nanolithography: A JEOL JSM-6400 scanning electron microscope with a Deben beam blanker is currently used for imaging and beam writing. The resolution of the electron beam at 40 kV is determined to be 3 nm, leading to a lateral resolution of 15 nm for imaging and writing. Fitted with Nabity software for lithography, the e-beam writer allows lithography and imaging on a variety of insulating and conducting substrates.
Substrate Prepapration: Spin-Coating, Surface Profiling, and Optical Lithography: The clean-room facility includes spin-coater, surface profilometer, high-resolution optical microscopes, ozone stripper, plasma cleaner and mask aligner for substrate preparation for lithography. For insulating structures, a thin conducting layer is deposited for high resolution e-beam lithography on substrate surface.
Material Deposition: Evaporators and Sputtering Systems: The facility includes three thermal evaporators for dedicated nonmagnetic deposition, and multiple-material deposition. In addition, there is a sputtering system for deposition of materials such as niobium, oxides and nitrites. The supporting optical processing facility (OPF) in Photonics Center also houses a number of thermal evaporators, e-gun evaporators, and sputtering chambers.
Structure Characterization: Characterization includes check for structural integrity, surface topography and electrical continuity of electrical lines. Nanofabricated structures are characterized with the JEOL scanning electron microscope (SEM), a digital instruments atomic force microscope (AFM), and a probe station. Electrical characterization is also performed at low temperatures in 4-Kelvin dippers.
Wirebonding, 4-Point Probe Station, Pulse Probe: The facility includes a wedge bonder and a thermal ball bonder for making electrical contacts between the nanoscale structures and contact pads. In some cases, a four-point probe station with precision current sources and LCR meter allows room temperature measurement without bonding. An Agilent oscilloscope with capabilities for time-domain reflection characterization is used to check for electrical continuity with picosecond pulses.
Additional Fabrication Facilities: The central support facility for fabrication is the optical processing facility (OPF), which houses a number of optical mask aligners, reactive ion etch system, e-gun evaporators, thermal evaporators, sputtering chamber, plasma cleaners, probe station, and other materials processing and characterization systems.
Ultra-Low Temperature Stealth Laboratory: Dilution Cryostat.
Our state-of-the-art stealth laboratory has been built specifically for the measurement of nanostructures in extremely sensitive environments. The laboratory provides unprecedented isolation from the entire spectrum of noise, from low-frequency vibration to high-frequency electromagnetic fields in the gigahertz range. The broadband isolation is achieved by suspending the entire concrete base of the laboratory, and constructing various levels of shielding, which, for the first time, incorporate stealth technology. Layers of RAM (radar absorbing material), copper floor and pit lining allow a minimum of 80 dB isolation of plane wave electric field up to 10 GHz. Two levels of concrete vibration isolation and suspension of the dilution fridge from an optical table on air legs minimize vibrations down to a level of sub-angstroms. Multiple levels of mu-metal shielding minimize the level of stray magnetic field down to the microgauss level.
COMPREHENSIVE DC-TO-DAYLIGHT NOISE ISOLATION DESIGN
i) Acoustic Noise: Three-Stage Acoustic Isolation: Acoustic noise (10 mHz – 10 kHz) arises because of the building vibration, seismic waves, ground noise due to nearby train or car movement, and the normal audible noise. The experimental setup in our lab — i.e. the sample inside the cryostat — has a three-level isolation: (i) acoustic room, (ii) suspension block from the building floor, and (iii) floating optical table. The cryostat hangs from the center of the optical table down inside a pit, which is designed to be an anechoic chamber. In addition, inside the cryostat, small suspensions with large spring constants are attached to further damp any mechanical vibration.
ii) Electromagnetic Noise: Three-Stage EMI Isolation: Electric field noise has a wide spectrum due to a variety of noise sources. Our experiments are sensitive at the level of 1.0e-18 watts or attowatts (electron decoherence requires on the order of 10 to 100 attowatts). The EMI (Electro-Magnetic Interference) noise sources are electrical 1/f noise (10 mHz – 10 kHz), MHz range RF noise (100KHz – 100 MHz), Low-Frequency microwave noise (10 MHz – 1 GHz) from microwave-powered instruments, cell-phone noise (0.1 – 10 GHz), and terahertz noise (sub-infrared noise due to thermal fluctuations, and optical noise (10 THz – 1.0e15 Hz).
In order to ensure the maximum attenuation or isolation of the noise figure over a broadband of frequencies the lab is designed to have a combination of multiple isolation mechanisms, including acoustic isolation. A specially-designed EMI-shielding room offers at least 100 dB isolation up to 1 GHz, and 80 dB isolation between 1 – 10 GHz. We also employ a Counter-Counter-Stealth Technology with nonmagnetic RAM (Radar-Absorbing Material) to selectively introduce additional attenuation in the TERAHERTZ range, following techniques developed to be a countermeasure for the terahertz-range Ultra WideBand (UWB) radars.
The EMI-shielding room has a thick-copper (non-magnetic) shielding of the floor and the pit to prevent any leakage of high-frequency sub-mm-wavelength electric fields. Additional filters are used to suppress the noise in high and low frequencies. Since we use both low-frequency transport and high-frequency reflection and transmission measurements, the filters are placed selectively to provide attenuation in the appropriate band. High-frequency filtering is obtained by Butterworth filters, and low-temperature silver-powder filters with typical attenuation of 80 dB at 1 GHz. For specific time-domain measurements, with complex bandwidth requirements, Gaussian filters such as Bessel-Thompson filters are used.
iii) Stray Magnetic Field Noise: Four-Stage Isolation: Without any magnetic shielding, the laboratory location was to found to have a RMS magnetic-field noise of 2 milligauss (per root hertz). This is primarily because of the close proximity of the building to the metro lines. In order to minimize the magnetic field noise to the micorgauss level, we have employed a series of magnetic isolation: mu-metal shielding around the dewar, non-magnetic copper EMI shielding by the shielding room, nonmagnetic pit, and a superconducting Nb shield around the sample.
iv) Thermal Noise Isolation: The measurements are carried out in a dilution cryostat capable of reaching temperatures down to 6 millikelvin (= 0.006 Kelvin). The low temperatures ensure the suppression of Johnson noise orders of magnitude below the required sensitivity for the measurement of ultrasensitive quantum effects (now determined by the first-stage preamplifier noise of 0.3 nV/rtHz at the required frequencies).
_
The two pictures on the left show the dilution cryostat (inside the blue dewar) hanging from an air-suspended optical table. The legs of the optical table stand on top of an isolated concreat pit with copper outlines both outside and on the top. The vibration-isolation pit is located inside the copper shielding room.
The three pictures on the right show the inside of the dilution cryostat insert, which is cooled in stages from room temperature to 4.2 Kelvin (by liquid helium), from 4.2 Kelvin to 1.5 Kelvin with a 1-Kelvin Pot (by pumping on helium), from 1.5 Kelvin to 0.750 Kelvin by condensing a mixture of helium-3 gas and helium-4 gas through a closed line, and from 0.750 Kelvin to 0.006 Kelvin by circulating the helium-3 gas in a closed cycle. The high-frequency coaxial cables at the end of the insert allow high-speed, high-frequency measurement of nanomechanical and nanoelectronics structures with unprecedented sensitivity. For example, the sensitivity is high enough to allow the detection of a circulating current due to ONE electron in a sub-micron ring, displacement due to the motion in a nanomechanical structure at the level of a femtometer, and damping or friction arising due to a handful tunneling atoms in a crystal.
Acoustic Laboratory for Nanomechanics
The acoustic lab has an acoustic shielding room designed to minimize noise from millihertz (vibration) to high megahertz (radio frequency) range. A three-level vibration isolation includes the acoustic shielding room, vibration-isolation pit and air-suspended optical table. A helium-3 cryostat hangs from the optical table, allowing a sensitive measurement environment down to 270 millikelvin in a field up to 9 tesla and frequencies up to 10 GHz.
The figures above and to the right show the top flange of the helium-3 cryostat fitted with high-frequency coaxial lines for measurements up to gigahertz range down to a temperature of 270 mK. Because of vibration noise isolation it is possible to detect the motion of nanomechanical structures which produce a displacement in the sub-picometer range (1.0e-14 m or 0.0001 angstrom). The coaxial lines allow high-frequency and short timescale measurements of the nanomechanical motion down to 40 ps in the time domain.
Bio-Functionalization Laboratory
A dedicated laboratory with a class-10,000 clean room is used for bio-functionalization processes. A nanoscale biosensor contains a force-detection element, which is typically a suspended nanomechanical structure. The other important part of the biosensor is the biosensing element, which is typically a nanoscale area on the nanomechanical structure, coated with a biomaterial for binding to a specific biomolecule. The aspect of selectively coating the nanomechanical sensor with biomaterial is called bio-functionalization. This lab contains a laminar-flow hood and a fume hood in addition to other instruments for the wet chemistry involved in bio-functionalization.